A basic knowledge of water movement is crucial to PCOs' understanding of how a termiticide behaves in soil and what factors influence its behavior. Understanding factors such as soil type and how water moves in saturated and unsaturated soil conditions will increase the PCO's overall knowledge of the challenges faced when applying a termiticide, and, coupled with some creative energy and imagination on the part of the PCO, will help pave the way toward overcoming those challenges during application.
Building construction intricacies aren't the only obstacles PCOs face when applying termiticides. Once a technician manages to get around all the structural challenges, the intricacies of the soil including water movement work their magic on the termiticide the PCO has just spent so much time applying.
In addition to soil characteristics such as pH and temperature, water movement in soil plays an important role in the distribution of termiticide. Although the movement of a termiticide through soil doesn't mimic water movement action-for-action, many of the same principles apply.
SOIL MAKEUP. In order to understand water movement in soil, it's important to know the basics of soil and its makeup. Soil is the loose surface of the earth consisting of not only solid particles, but water and air as well. The solid particles are usually less than 2 millimeters in diameter, and are categorized based on their diameter size:
• Sand 0.05 to 2 millimeters.
• Silt 0.002 to 0.05 millimeters.
• Clay less than 0.002 millimeters.
The relative proportions of sand, silt and clay are used to define the texture of the soil as a coarse or fine (heavy) soil. Sandy loam is a coarse soil, whereas clay loam is a fine soil.
When water is applied to the ground's surface as rain or irrigation, or during a termiticide application, it either moves into the soil or evaporates into the atmosphere. The manner in which water or termiticides move through soil is dependent primarily on the properties of the soil, the interaction between water and the soil, the soil moisture gradient, and the changes in soil properties with depth.
The rate of water movement and the depth or distance that water can move are influenced by the size, numbers and continuity of soil pore spaces. The spaces between solid particles are voids, or pore spaces, which contain water and air. The pore space sizes are larger in a soil composed mainly of large solid particles, such as sand, than in a soil composed of finer soil particles, such as clay.
Even though a fine soil has smaller soil pores than a coarse soil, it has a much greater number of pore spaces than a coarse soil. Therefore, water as well as termiticide solutions tend to move more slowly through a fine soil such as clay loam than through a coarse soil such as sand. As water moves into the pore spaces, it displaces the air while filling the pores with water. If the pore spaces are blocked by entrapped air bubbles, the continuity of pore spaces to conduct water is broken. Water movement is reduced through these discontinuous pores.
Water movement through soil pores is further influenced by the interaction of water with the solid soil particles, which are composed mainly of mineral materials and a small percentage of organic materials. The water moving through the soil pore spaces is similar to water moving through a capillary tube.
The adhesion or capillary forces bind water molecules to the surfaces of solid particles. The smaller the pore spaces, as in the case of clay loam, the more tightly the capillary water is adhered to the soil surfaces and will not move readily in soil. Cohesion forces keep the water molecules together in the pore spaces, thus forming localized bodies of free-standing water within the pore spaces. Known as gravitational water, this type of water flows readily through soils. Cohesive forces also assist the movement of a termiticide in the soil.
UNSATURATED WATER FLOW. When the soil pore spaces are partially filled with water, the soil is in an unsaturated moisture condition. Unsaturated water flow is slow, and occurs mainly by adjusting the thickness of water films, or capillary water that surround the soil particles. Adjusting the thickness of water surrounding the solid surfaces is like water moving up a capillary tube or a drop of water spreading out on a piece of glass. Water in unsaturated soil tends to have little movement.
The soil moisture gradient, which is the difference in the water content from one soil zone to another, is the driving force for unsaturated water flow. Water flows from pores in wetter soil zones to pores in drier soil zones. Water under unsaturated conditions can move downward, horizontally or upward, depending on the position of the drier soil zone in relation to the wetter soil zone.
SATURATED WATER FLOW. Soil is considered saturated when all pore spaces are filled with water. Saturated water flow is rather rapid, and occurs mainly by draining the gravitational water occupying the pore spaces between the soil particles.
The size of the soil pores is the main influence on saturated water flow. Like a capillary tube, the soil pores should be continuously connected to each other in order to form a conduit for water to move through the soil. Coarse soil has larger pores, enabling it to conduct saturated water flow faster than fine-textured soil.
Gravitational forces are the main driving force for saturated water flow. The direction of saturated water flow is usually downward. Horizontal, or lateral, water flow in soil under saturated conditions occurs slowly because the force of gravity doesn't assist horizontal water flow. Upward saturated water flow is very limited because the force of gravity hinders the upward flow. Termiticides are subject to the same movement in soil.
COMBINED FLOW. In soils, saturated water flow occurs less frequently than unsaturated water flow. Soil saturation occurs when soil surfaces are flooded by heavy rain or irrigation application, at lower horizons of poorly drained soils, and at portions of well-drained soil lying above stratified layers of heavier soil types.
Saturated soil zones are usually localized, and may occur in close proximity to unsaturated soil zones. For example, water puddles, which form on the soil surface after heavy rain, may lie just a few centimeters from an unsaturated zone below the soil. Another example is the area underneath a downspout. The soil underneath the downspout will stay very saturated, but moving just six inches either way, the soil would be very dry and unsaturated. In these situations, the saturated water flow becomes unsaturated water flow as it moves from the saturated zone into the unsaturated zone.
When rodding, the termiticide is subject to saturated flow around the specific rodding area. Then, as the solution moves out into unsaturated areas, the termiticide would begin to move more slowly.
The rate at which water moves in soil depends on gravity and the soil moisture gradient between the saturated soil zone (water front) and the unsaturated (drier) soil beneath. Once drained of its gravitational water, further water movement in the previously saturated soil will depend on the forces of unsaturated water flow.
Lateral and upward movements of termiticide solutions in soils that are fully saturated with water are very limited. However, when soils are under unsaturated conditions, termiticide solutions have a greater tendency to move laterally and even upward toward the soil surface. Water movement facilitates the distribution of termiticide from the application sites (rod holes) to the surrounding soil.
RESTRICTIONS TO WATER FLOW. Soil under natural conditions has layers or horizons with differing textural and structural properties. For example, during pre-construction treatments, topsoil most likely was placed over a sand or clay base. A compacted fine-textured soil layer will be almost impermeable to water flowing from a coarser soil layer above it. Likewise, water flow from a fine-textured soil (clay loam) to the adjacent layer of coarse-textured soil (sand) will be restricted at the interface of the two soils.
The sand layer has large pores and low adhesive forces for water. Clay loam has small pores and greater adhesive forces for water, thus holding the water film tightly to the clay loam surfaces. Water in the clay loam layer will not be pulled down into the sand layer until the water content within the pores of the clay loam is high enough to overcome the adhesive forces that hold the water to the soil surfaces. During this time, downward water movement is temporarily impeded.
Likewise, the movement of applied termiticide solution can be temporarily halted at the boundary between the topsoil later and the lower layers of different soil textures. For this reason, the assumption can't be made that the termiticide will move rapidly past the topsoil layer to lower soil depths.
When enough gravitational water is built up at the interface of the two layers, downward water flow will resume, and water will move into the larger pores of the sand layer. Consequently, the clay loam layer above the sand layer will become nearly saturated before the water continues to move down into the sand layer. During the temporary cumulation of water at the interface of the soil horizons, water in the clay loam soil horizon will have a greater tendency to move laterally by capillary action along the interface, moving from wetter to the adjacent drier soil zones.
Water movement is just one of several factors that influence the performance of a termiticide once it is placed in the soil. Applying the correct, label-specified amount of termiticide solution is crucial to ensuring adequate movement and distribution through the soil. At the same time, applying too much termiticide or making an application against what the soil conditions dictate will leave the termiticide solution in the hands of Mother Nature.
Pau Yun, Ph.D., is a research chemist with Miles Inc.'s Agriculture Division Environmental Research Section. Some
information in this article is derived from The Nature and Properties of Soils, 10th Edition, Brady, N.C. 1990.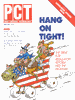
Explore the April 1995 Issue
Check out more from this issue and find you next story to read.
Latest from Pest Control Technology
- Los Angeles Leads Orkin’s 2024 Top Mosquito Cities List
- The Industry’s First Line of Defense: Protecting the Perimeter
- Golden Circle Exterminators Celebrated 50th Anniversary
- Global Pest Management Coalition Announces World Pest Day 2024 Celebration
- Orkin Helps Local Youth Sports Program Design a ‘Killah’ New Logo
- ABC Home & Commercial Services Celebrates 75 Years in Business
- NPMA Academy Announces Full Schedule
- Truly Nolen Promotes Marchello, Christopherson and Bolton to Managerial Positions